Manipulating Synaptic Networks using Optogenetics and Mathematical Modelling
Akshita Jindal, 1st year PhD, University of Exeter
BACKGROUND:
​
The brain is a complex puzzle. All of our behaviours, such as recognising friends or making a cup of tea, result from the coordinated behaviour of networks of neurons in our brain. Each behaviour is characterised by a unique pattern of electrical activity within these neural networks. Understanding the origins of these activity patterns is one of the key challenges in the field of neuroscience.
​
My profound fascination lies in the innovative field of neuroscience that combines the power of optogenetics with mathematical modeling, to unravel the intricate workings of synaptic networks in the brain. Optogenetics, a multidisciplinary approach, involves genetically encoded proteins called opsins to control and manipulate the activity of targeted cells using light. However, the complexity of the brain demands more than just experimentation; it requires a mathematical framework. Mathematical modelling complements optogenetics by providing a structured language to describe and predict synaptic interactions.
​
These mathematical models empower us to simulate and understand the emergent properties of neural networks, unveiling the hidden patterns and principles governing brain function. The implications of my work extend far beyond the laboratory, ranging from mapping neural circuits, peering into the neural underpinnings of behaviour, and potentially guiding therapeutic interventions for neurological and psychiatric disorders.
​
METHODOLOGY:
​
I am currently using the GT1-7 mouse hypothalamus cell line to record electrical activity from the cells expressing two distinct types of opsins: channelrhodopsin-2 (ChR2), which has an excitatory effect, and Halorhodopsin (eNpHr2.0), an inhibitory opsin. These recordings are done using patch-clamp electrophysiology. The primary objective of this endeavour is to understand the variation of photocurrents that are generated on specific light stimuli. Additionally, it will help to establish an experimental setup that will facilitate precise optogenetic manipulation within neural networks.
​
RESULTS:
​
ChR2 is activated by the light of wavelength 470nm. It initiates cell depolarisation through an influx of positively charged ions. Conversely, eNpHr3.0 serves as a chlorine ion pump and induces hyperpolarisation by promoting the influx of negatively charged chloride ions. These distinctive responses are quantified through photocurrent measurements conducted via voltage-clamp experiments, yielding results as illustrated in the figure below:
​
a) b)
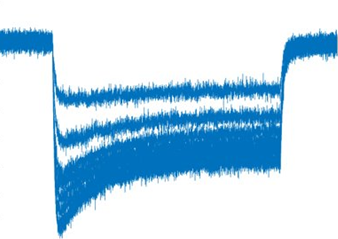
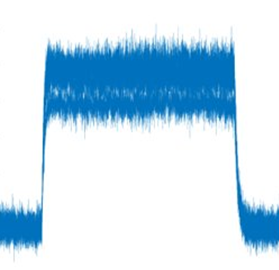

Fig 1. The variations in photocurrent on stimulation with different light intensities (a) ChR2 and (b) eNpHr2.0
​
Fig 2. The GT1-7 cells transfected with eNphr3.0 (red) and ChR2 (green) opsins
FUTURE WORK:
​
Having explored the intricacies of photocurrent variations, my near future research plan is to simultaneously record and visualise the electrical activity using fluorescence reporters such as EGFP and mCherry, employed within the opsins. This multifaceted approach promises a comprehensive understanding of neural activity, enriching our insights at both the electrophysiological and optical levels.
​
FUNDED BY:
​
​
​
CONTACT:
​
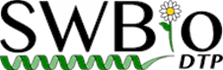

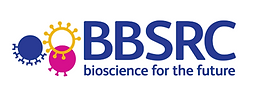
@AkshitaJindal__